BAQIS Internal Seminar 13: Theoretical design for Si quantum materials
2020/06/05
Date: 5-Jun-2020
Time: 14:00-15:00
Venue: Meeting Room 526
Speaker: Prof. Junwen LUO (Division of Quantum Materials and Devices)
Abstract:
Investments of trillions of dollars in equipment and research and development activities during the past half-century have made the Si technology very mature and created a powerful industrial capacity. That suggests Si will be completely replaced is unlikely to happen anytime soon and Si will still play a prominent role well into the foreseeable future. However, Moore's law, which has been used in the semiconductor industry to guide long-term planning and to set targets for research and development, is approaching physical limits. The integration of optoelectronic devices and quantum devices on Si microelectronics chips is a feasible way to overcome the physical limits to transistor scaling. In this talk, we will present our recent works on Si-based light emitting and energetic valley splittings in SiGe heterostructures, which are two key issues for developing optoelectronic integration circuits and Si quantum computation. We have theoretically inverse designed Si quantum well to isolating a single electron valley state in Si by a magic-sequence of Ge/Si barrier layers, giving rise to a valley splitting as large as 9 meV. We found the Si Rashba spin-orbit coupling is enhanced by a factor of 2000 in holes of one-dimensional Si nanowires in comparison with two-dimensional electron gases and reaches a value even larger than that of InAs two-dimensional electron gases. Therefore, we solve the issue of Si having a so weak spin-orbit coupling effect. We also design Si/Ge magic sequence superlattices exhibit orders more efficient at emission light than their existing counterpart records and approach more than 10% brightness of real direct gap materials, such as GaAs. Furthermore, regarding the direct bandgap is only 140 meV above the indirect bandgap in Germanium (Ge), it is well established that a tiny (1-2%) extensile strain could render an indirect-to-direct bandgap transition and makes it direct bandgap. However, there is no feasible way to create such extensive strain on Ge since the lattice parameter of Ge is even 4.3% larger than Si. We propose insert external atoms into interstitial sites of Ge, which grows on Si substrate through SiGe buffer layer, to induce an effective tensile uniaxial strain on Ge, a new pathway toward direct band gap Ge. Because our proposed scheme is fully compatible with CMOS technology, our new pathway would be a solution for on-chip lasers.
Abstract:
Investments of trillions of dollars in equipment and research and development activities during the past half-century have made the Si technology very mature and created a powerful industrial capacity. That suggests Si will be completely replaced is unlikely to happen anytime soon and Si will still play a prominent role well into the foreseeable future. However, Moore's law, which has been used in the semiconductor industry to guide long-term planning and to set targets for research and development, is approaching physical limits. The integration of optoelectronic devices and quantum devices on Si microelectronics chips is a feasible way to overcome the physical limits to transistor scaling. In this talk, we will present our recent works on Si-based light emitting and energetic valley splittings in SiGe heterostructures, which are two key issues for developing optoelectronic integration circuits and Si quantum computation. We have theoretically inverse designed Si quantum well to isolating a single electron valley state in Si by a magic-sequence of Ge/Si barrier layers, giving rise to a valley splitting as large as 9 meV. We found the Si Rashba spin-orbit coupling is enhanced by a factor of 2000 in holes of one-dimensional Si nanowires in comparison with two-dimensional electron gases and reaches a value even larger than that of InAs two-dimensional electron gases. Therefore, we solve the issue of Si having a so weak spin-orbit coupling effect. We also design Si/Ge magic sequence superlattices exhibit orders more efficient at emission light than their existing counterpart records and approach more than 10% brightness of real direct gap materials, such as GaAs. Furthermore, regarding the direct bandgap is only 140 meV above the indirect bandgap in Germanium (Ge), it is well established that a tiny (1-2%) extensile strain could render an indirect-to-direct bandgap transition and makes it direct bandgap. However, there is no feasible way to create such extensive strain on Ge since the lattice parameter of Ge is even 4.3% larger than Si. We propose insert external atoms into interstitial sites of Ge, which grows on Si substrate through SiGe buffer layer, to induce an effective tensile uniaxial strain on Ge, a new pathway toward direct band gap Ge. Because our proposed scheme is fully compatible with CMOS technology, our new pathway would be a solution for on-chip lasers.
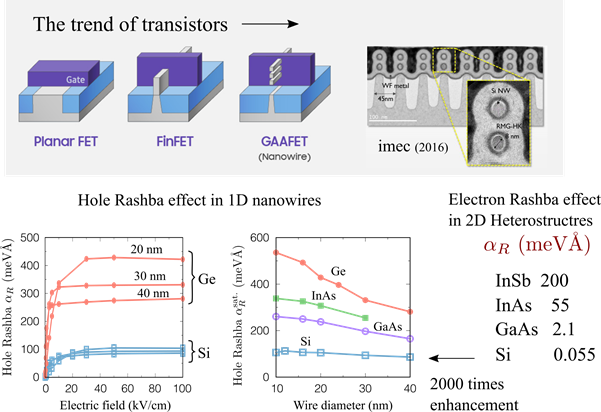
Date: 5-Jun-2020
Time: 14:00-15:00
Venue: Meeting Room 526
Speaker: Prof. Junwen LUO (Division of Quantum Materials and Devices)
Abstract:
Investments of trillions of dollars in equipment and research and development activities during the past half-century have made the Si technology very mature and created a powerful industrial capacity. That suggests Si will be completely replaced is unlikely to happen anytime soon and Si will still play a prominent role well into the foreseeable future. However, Moore's law, which has been used in the semiconductor industry to guide long-term planning and to set targets for research and development, is approaching physical limits. The integration of optoelectronic devices and quantum devices on Si microelectronics chips is a feasible way to overcome the physical limits to transistor scaling. In this talk, we will present our recent works on Si-based light emitting and energetic valley splittings in SiGe heterostructures, which are two key issues for developing optoelectronic integration circuits and Si quantum computation. We have theoretically inverse designed Si quantum well to isolating a single electron valley state in Si by a magic-sequence of Ge/Si barrier layers, giving rise to a valley splitting as large as 9 meV. We found the Si Rashba spin-orbit coupling is enhanced by a factor of 2000 in holes of one-dimensional Si nanowires in comparison with two-dimensional electron gases and reaches a value even larger than that of InAs two-dimensional electron gases. Therefore, we solve the issue of Si having a so weak spin-orbit coupling effect. We also design Si/Ge magic sequence superlattices exhibit orders more efficient at emission light than their existing counterpart records and approach more than 10% brightness of real direct gap materials, such as GaAs. Furthermore, regarding the direct bandgap is only 140 meV above the indirect bandgap in Germanium (Ge), it is well established that a tiny (1-2%) extensile strain could render an indirect-to-direct bandgap transition and makes it direct bandgap. However, there is no feasible way to create such extensive strain on Ge since the lattice parameter of Ge is even 4.3% larger than Si. We propose insert external atoms into interstitial sites of Ge, which grows on Si substrate through SiGe buffer layer, to induce an effective tensile uniaxial strain on Ge, a new pathway toward direct band gap Ge. Because our proposed scheme is fully compatible with CMOS technology, our new pathway would be a solution for on-chip lasers.
Abstract:
Investments of trillions of dollars in equipment and research and development activities during the past half-century have made the Si technology very mature and created a powerful industrial capacity. That suggests Si will be completely replaced is unlikely to happen anytime soon and Si will still play a prominent role well into the foreseeable future. However, Moore's law, which has been used in the semiconductor industry to guide long-term planning and to set targets for research and development, is approaching physical limits. The integration of optoelectronic devices and quantum devices on Si microelectronics chips is a feasible way to overcome the physical limits to transistor scaling. In this talk, we will present our recent works on Si-based light emitting and energetic valley splittings in SiGe heterostructures, which are two key issues for developing optoelectronic integration circuits and Si quantum computation. We have theoretically inverse designed Si quantum well to isolating a single electron valley state in Si by a magic-sequence of Ge/Si barrier layers, giving rise to a valley splitting as large as 9 meV. We found the Si Rashba spin-orbit coupling is enhanced by a factor of 2000 in holes of one-dimensional Si nanowires in comparison with two-dimensional electron gases and reaches a value even larger than that of InAs two-dimensional electron gases. Therefore, we solve the issue of Si having a so weak spin-orbit coupling effect. We also design Si/Ge magic sequence superlattices exhibit orders more efficient at emission light than their existing counterpart records and approach more than 10% brightness of real direct gap materials, such as GaAs. Furthermore, regarding the direct bandgap is only 140 meV above the indirect bandgap in Germanium (Ge), it is well established that a tiny (1-2%) extensile strain could render an indirect-to-direct bandgap transition and makes it direct bandgap. However, there is no feasible way to create such extensive strain on Ge since the lattice parameter of Ge is even 4.3% larger than Si. We propose insert external atoms into interstitial sites of Ge, which grows on Si substrate through SiGe buffer layer, to induce an effective tensile uniaxial strain on Ge, a new pathway toward direct band gap Ge. Because our proposed scheme is fully compatible with CMOS technology, our new pathway would be a solution for on-chip lasers.
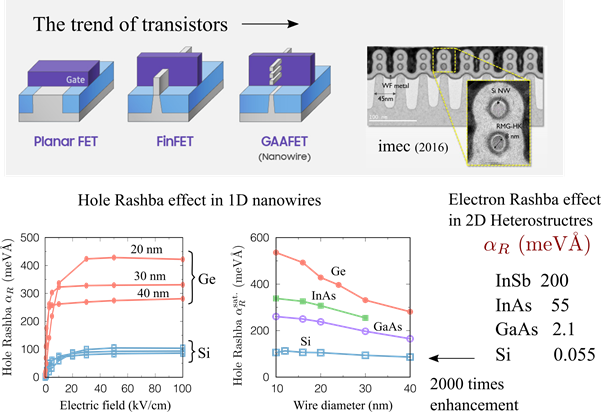
About the speaker:
Jun-Wei Luo is a professor of Institute of Semiconductors, Chinese Academy of Sciences and serving as a deputy director of State Key Laboratory of Superlattices and Microstructures. He is also an adjoint professor of Beijing Computational Science Research Center. In 2019, he rewarded the National Science Foundation for Distinguished Young Scholars. He received his Bachelor and Master degrees from the Zhejiang University in 2000 and 2003, respectively, and Ph.D in condensed matter physics from the Institute of Semiconductors, Chinese Academy of Sciences in 2007. Before moving back to China in 2014, he associated the U.S. national renewable energy laboratory (NREL) as a postdoc, permanent position staff, and then senior scientist. He focuses mainly on design of Silicon-based light emitting and spin-orbit coupling effect in semiconductors, towards the realization of optoelectronic integration circuits (OEIC) and semiconductor quantum integration circuits, respectively. He has published more than 90 peer-reviewed papers. Specifically, since returning to China from US National Lab in 2014, he as the first author and/or corresponding author has published one paper in nature physics, one in nature nanotechnology, two in PRL, and one in nature communications. He was invited to talk or serviced as a session chair in prestigious international conferences such as APS, ACS, E-MRS, JSAP-MRS, and ICSNN